Curious about which LC column type is best for your application?
Join our free webcast on Thursday 26th June at 3pm BST to explore Fully vs Superficially Porous Particles - their pros, cons, and when to use each for optimal performance and sustainability.
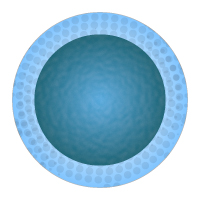
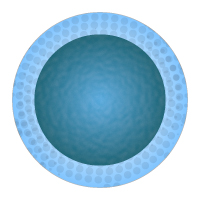
To use a fully porous particle or to use a superficially porous particle? What are the differences and why do they make a difference?
Since the introduction of high-performance liquid chromatography (HPLC) nearly 55 years ago, major advancements in column stationary phases have significantly improved separation speed and efficiency. Traditionally, fully porous particles (FPP), composed entirely of porous silica with variable pore volumes have been the standard. In the 1970’s, building on the work of Casba Horvath and co-workers, Jack Kirkland, then working at the Du Pont Company, developed superficially porous particle technology (SPP) (also called core–shell, fused-core -, partially porous or solid-core), beads with 30 µm glass cores with layers of silica sol deposited on the surface. These materials were developed until, in 2006, Advance Material Technology (AMT) introduced the HALO SPP range featuring a solid silica core with a porous outer shell of Type B silica sol, and these and similar materials have further developed and are now offered by a range of manufacturers, (e.g. Agilent Poroshell and Thermo Scientific Accucore) offering more uniform and shallower pore structures [1].
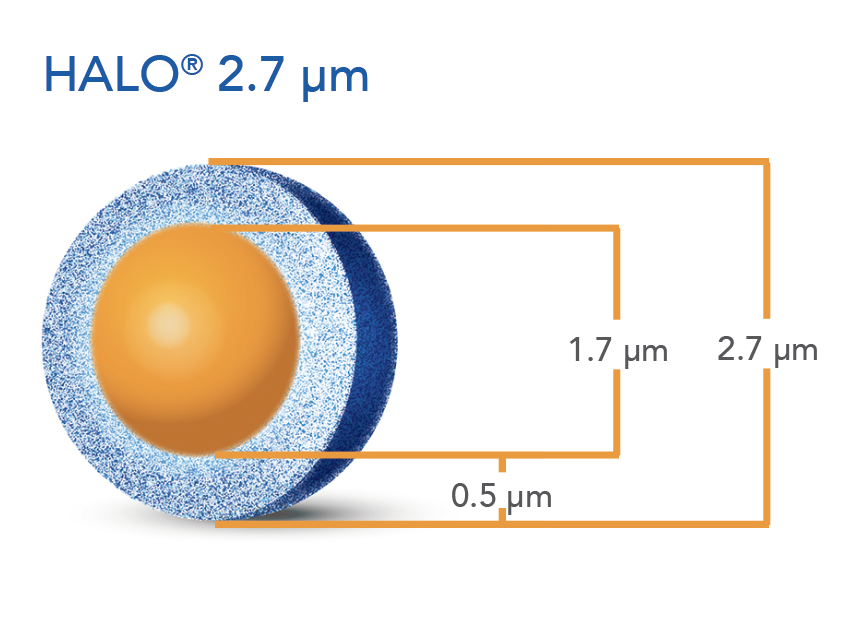
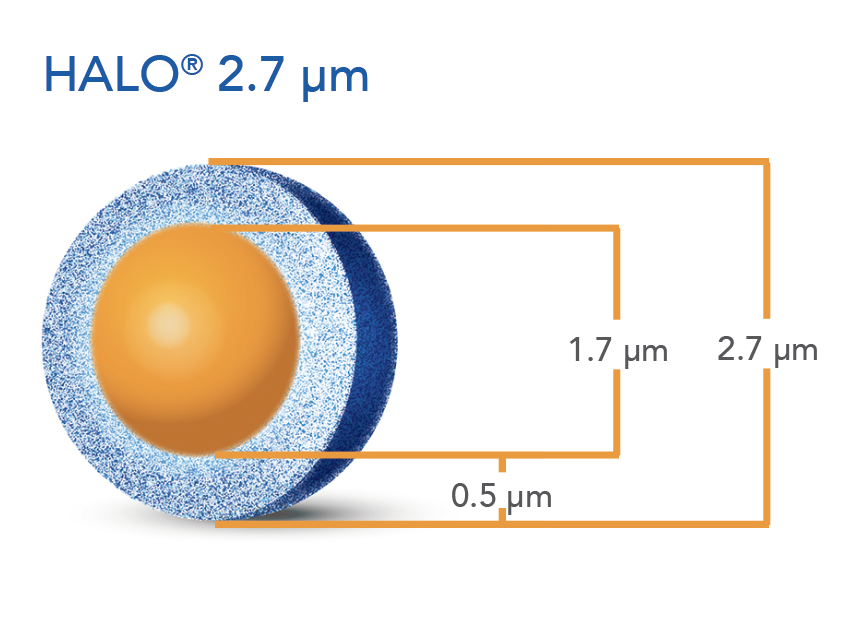
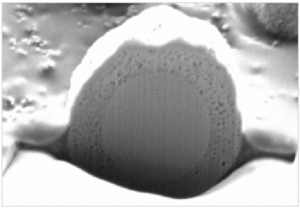
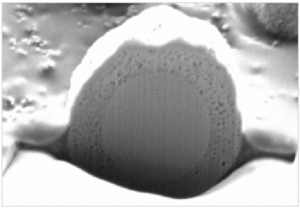
Figure 1. SEM image of first commercial HALO® particle with 2.7 µm total size consisting of a 1.7 µm solid silica core and a 0.5 µm shell [2]
Key strategies driving performance improvements include increased packed-bed homogeneity, reducing intra-particle volume, narrower particle size distribution, and minimised diffusion distances using smaller or superficially porous particles.
Both FPP and SPP columns have their merits depending on analytical requirements. FPP have been the tried and tested option for over 55 years and given the new advances in ultra-high performance liquid chromatography (UHPLC), using smaller (typically sub-2 µm) particles, these columns can provide extremely efficient, and sensitive analyses. As described above, the widespread use of modern SPP columns is a more recent offering for providing efficient and sensitive analysis.
Advancements in Fully Porous Particles
Fully porous particle columns with 5 µm particle sizes are associated with broader peak profiles, reduced resolution and lower sensitivity and these limitations are especially evident when compared to columns packed with 3 µm or sub-2 µm particles. The primary performance advantage of FPP columns lies in the use of these smaller particle sizes, which should be considered standard when selecting FPP technology for new method development. This is supported theoretically by the enhanced efficiency observed with decreasing particle size (Figure 2). Lower values of H (the height equivalent to a theoretical plate, or plate height) equate directly to increased chromatographic efficiency and hence inherently narrower peaks).
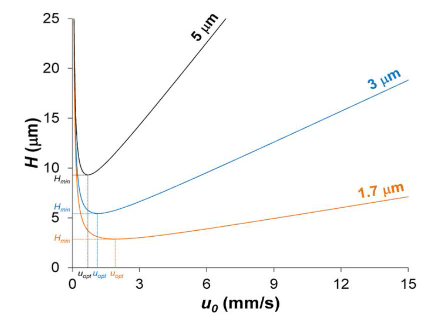
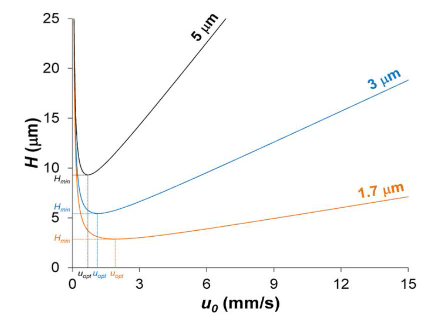
Figure 2. Theoretical relationship between particle size and column efficiency as described by the van Deemter equation [3]
Further, the reduction in plate height associated with smaller particles broadens the range of optimal mobile phase linear velocities (or flow rates at a fixed column internal diameter), enabling higher flow rates without compromising column efficiency. This gives the end user the choice of performing more complex separations in a similar time frame or equivalent separations in a shorter time frame, compared to the 5mm particle sizes.
Whilst a detailed discussion on the mechanism of increased efficiency for smaller FPP’s is beyond the scope of this article, it is widely accepted that reducing particle size in chromatographic columns enhances packing uniformity, promoting a more homogeneous and monodisperse bed structure. Smaller particles inherently exhibit a narrower particle size distribution, which minimizes interstitial void variability and reduces eddy diffusion. As a result, analyte pathways through the column become more consistent and linear, decreasing the height equivalent to a theoretical plate (HETP) and increasing column efficiency. Chromatographically this manifests as reduced peak width and reduced analysis time, as described above.
The example below, from Agilent, demonstrates how altering the particle size of FPP columns from 5µm to 1.8 µm can significantly reduce the analysis time while still producing narrow and well resolved peaks (Figure 3) [4]. The chromatograms show that by reducing the particle size of the column the same analysis can be achieved in a reduced time (A) run time >11minutes whereas (B) showed a run time of 5 minutes, while still achieving baseline resolution for all compounds. The reduction in particle size also meant a shorter column was able to be implemented reducing the length from 150 mm (A) to a 50 mm (B), this will also help minimise longitudinal diffusion.
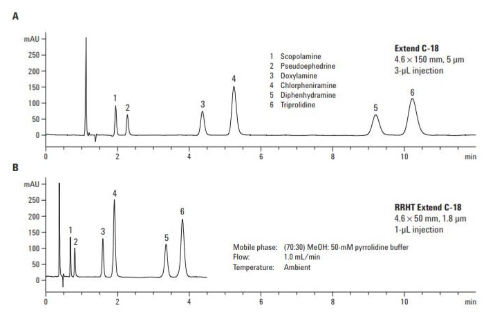
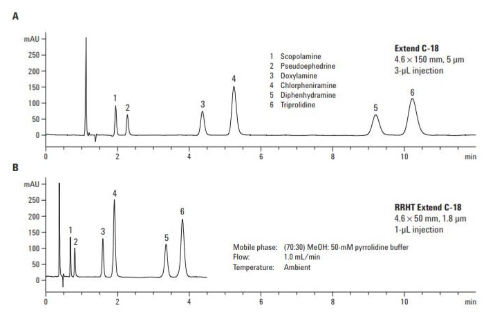
Figure 3. Assay of antihistamines at high pH on Agilent Extend C18, (A) 4.6 x 150 mm 5 µm (B) 4.6 x 50mm 1.8 µm [4]
One of the most significant advancements in FPP column technology has been the introduction of hybrid silica materials, which integrate both organic and inorganic moieties within the silica matrix. Hybrid silica particles provide enhanced mechanical and chemical stability, enabling operation across broader pH ranges and elevated temperatures [5]. The robustness and versatility of hybrid silica columns have been further refined by manufacturers such as YMC, whose Triart series incorporates hybrid silica with advanced multistage end-capping. Unlike conventional end-capping, which typically employs a single reagent and leaves a substantial proportion of surface silanols unreacted, multistage end-capping utilizes multiple reagents with varying reactivity to more effectively ‘cap’ a higher proportion of residual silanol groups. This significantly reduces silanol activity, improving column stability, reproducibility, and reducing unwanted secondary interactions [6], leading to improved peak shape and improved analytical reproducibility.
The widespread adoption of this column technology has led to a broad range of phase options, providing broad selectivity options for method development where the use of high or low pH as well as elevated temperature can be utilised to affect the separation (Figure 4). Continuing the advancements in FPP technology, hybrid silica columns are available in a range of particle sizes from 5 µm (and larger for preparative-scale applications) to sub-2 µm particles enabling compatibility with both conventional HPLC and UHPLC systems.


Figure 4. YMC Triart phase overview [6]
The primary limitation of reduced particle size in FPP columns is the associated increase in system back pressure, which can offset the efficiency gains of 3 µm and sub-2 µm particles and restrict widespread replacement of conventional 5 µm particle methods. This elevated pressure demand renders their use impractical for systems lacking sufficient pressure tolerance. Specifically, sub-2 µm particles necessitate ultra-high-performance liquid chromatography (UHPLC) systems rated to 1000bar or higher, while 3 µm particles typically require HPLC systems rated to pressures up to 600 bar.
The relationship between back pressure and particle diameter can be defined by the Poiseuille equation (equation1) which shows the significant influence on pressure is the packing particle diameter dp. as it is inversely proportional to dp2. Decreasing the particle size by half increases the pressure by a factor of 4. However, by reducing column length (L) can help mitigate some of the pressure increase without negatively effecting the resolution too much [7].
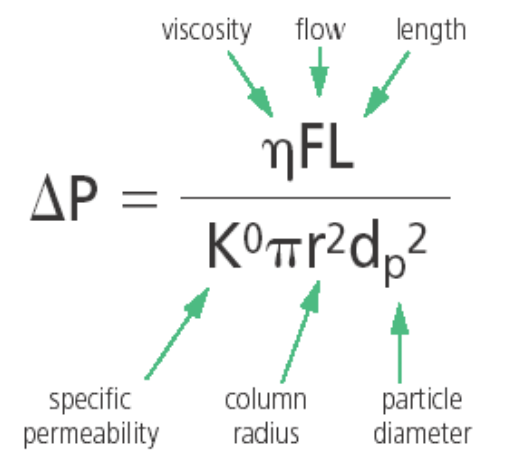
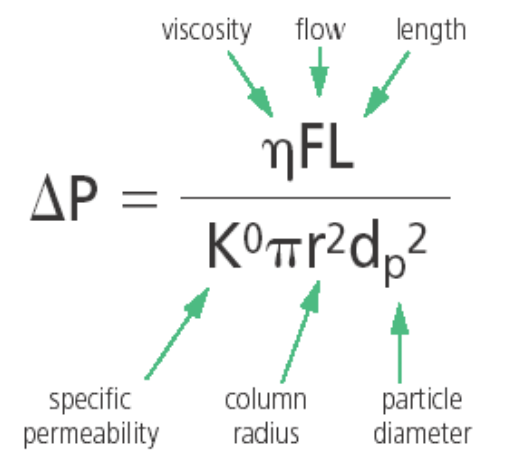
Equation 1. Poiseuille Equation [7]
Another limitation worth considering with reduced particle sizes, particularly sub 2 µm particles is the need to use low dispersion systems. It is difficult to see the efficiency gains of sub 2µm particles if the diffusion volume of the system is not carefully controlled and minimised as this results in an increase in longitudinal diffusion which can offset any peak width reductions gained from sub 2 µm particles.
The rise of Superficially Porous Particles
Superficially porous particle columns, as previously stated, are relatively newer and less widely adopted among chromatographers, especially those using older pharmacopeial methods for example. SPP technology offers advantages which address several limitations associated with reduced particle size FPP columns, the main benefit being improved efficiency at lower system back pressures due to their core-shell structure.
Manufacturers have stated SPP columns can achieve efficiencies comparable to sub-2 µm FPPs, but with significantly lower back pressure, thus eliminating the need to purchase costly UHPLC systems. As discussed, a major limitation of sub-2 µm FPP columns is the elevated pressure requirement, often exceeding standard HPLC capabilities. In contrast, SPP columns can deliver high efficiency while operating at pressures ≤600 bar. The Van Deemter curves shown in Figure 5 further illustrate the potential of SPPs to outperform sub-2 µm FPPs under certain analytical conditions, making them an attractive option for chromatographers wanting to achieving rapid, sensitive, and high-resolution separations.
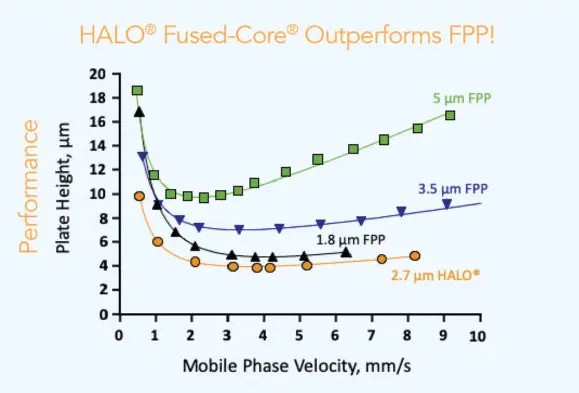
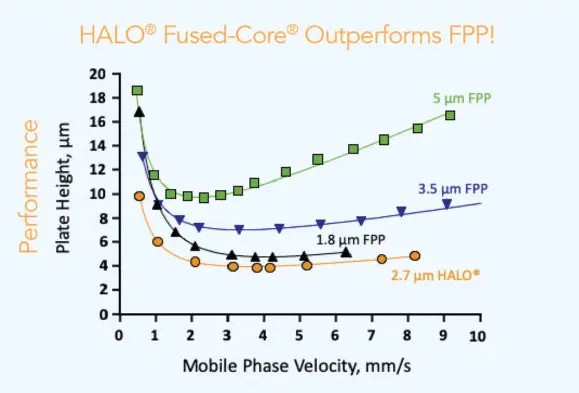
Figure 5. Van Deemter curve for various fully porous particles and for 2.7 µm superficially porous particle, assessed under the following conditions - 4.6 x 50 mm, 24 °C; 40/60 water/ACN; Solute: naphthalene [2]
The Van Deemter plot plays a critical role in assessing column performance, as previously mentioned with FPP, increased homogeneity of the column packing results in a reduction in eddy diffusion and produces enhanced efficiency. This effect is also true for SPP columns, if not further improved as demonstrated in Figure 6, due to the tightly controlled manufacturing process for creating core-shell particles. The process involves the manufacture of the solid silica core to an accurate diameter followed by a controlled superficial layer deposition which is built up in layers to ensure the desired thickness is achieved and consistent.
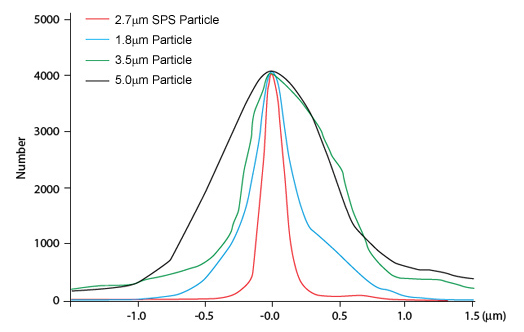
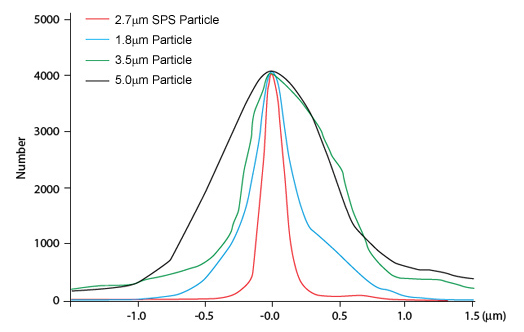
Figure 6. Particle size distribution of different SPPs and FPPs [8]
A reduction in mass transfer is typically exhibited with SPP columns due to a reduction in pore depth compared to FPP. The shortened diffusion path within the porous shell facilitates more rapid analyte mass transfer between the mobile and stationary phases, enabling faster elution and improved peak efficiency. The enhanced mass transfer kinetics contribute to analysis times that are comparable to those achieved with UHPLC methods, despite operating at lower system pressures.
Transitioning from a 5 µm FPP column to a 2.7 µm SPP column demonstrates the efficiency gains achievable with modern SPP technology. As shown in Figure 7, translating a USP Lansoprazole method to a 150 × 4.6 mm SPP column from a 150 x 4.6 mm FPP column, improved resolution from 1.78 to 2.38 and reduced run time from over 30 minutes to under 20 minutes, highlighting the superior performance of SPPs.
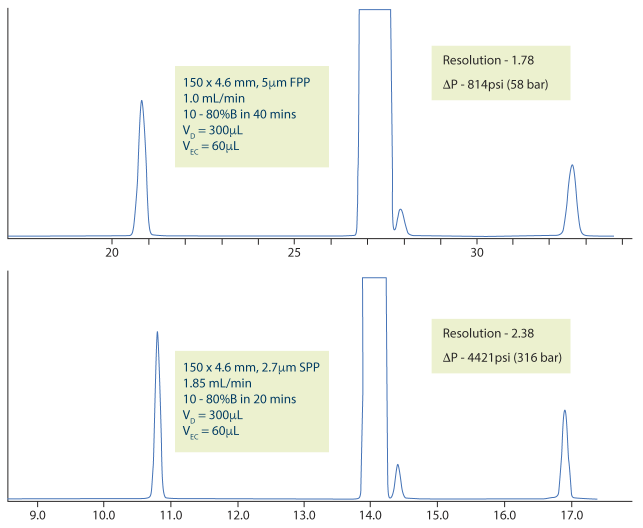
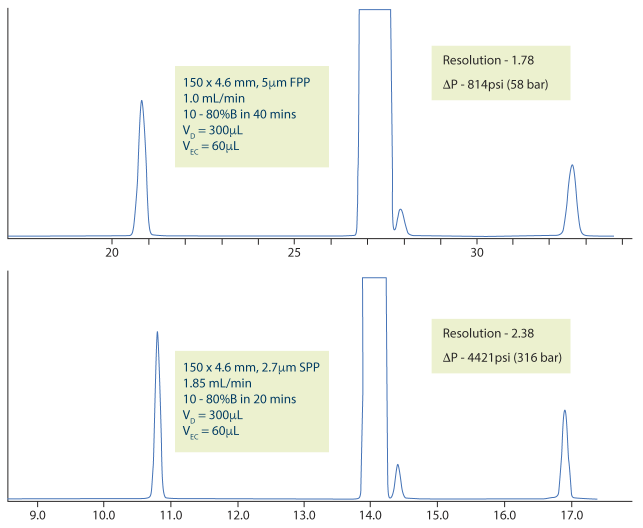
Figure 7. Original USP method for Lansoprazole impurities (top) same method employed on a 2.7µm SPP column with th esame dimensions (bottom) [9]
Method development of mobile phase flow rates, gradients and injection volume alterations can lead to a successful FPP to SPP method transfer however selectivity equivalence must be carefully evaluated, as differences can arise even between columns, even from the same manufacturer. Notably, Agilent has demonstrated strong selectivity consistency between its Zorbax (FPP) and Poroshell (SPP) columns, as evidenced by the successful method translation shown below.


Figure 8. Method transfer from a FPP Agilent Zorbax Eclipse Plus C18, 4.6 x 250 mm 5 µm to a SPP Agilent InfinityLab Poroshell 120 EC-C18, 4.6 x 100 mm 2.7 µm column [10]
The advantages of SPP columns over larger particle FPP columns are evident due to reduced analysis times, enhanced peak response, and improved efficiency (and hence resolution). While method transfer from FPP to SPP columns is achievable, it often requires method optimisation and scaling translation calculations. These adjustments can be minimized or avoided altogether by incorporating SPP columns during initial method development, especially given that many manufacturers now offer SPP phases designed to closely match traditional FPP chemistries.
Examples:
AMT HALO phases: C18 (Aq and PCS), C8, Biphenyl, Phenyl-hexyl (PCS), PFP, CN, LPH-C18 and RP-Amide
Agilent Poroshell: C18 (Aq and CS), SB C18, SB C8, C8, Phenyl-hexyl, PFP, CN, HPH-C18 and Bonus-RP
As with FPP columns, there are limitations associated with SPP columns which need to be considered when making the choice between these stationary phase particles. The primary concern is their reduced loading capacity relative to FPP columns, attributable to a lower overall surface area illustrated in Figure 1. While approximately 75% of an SPP's volume is porous, this results in a limited decrease in loadability. However, this limitation can typically be mitigated through minor method adjustments, such as reducing analyte concentration or injection volume and sensitivity is often maintained due to the inherent increase in efficiency (and hence signal to noise) when switching between FPP and SPPs.
Summary
Both particle types have their place in chromatography whether you have UHPLC capabilities and want to capitalise on this with sub 2µm FPP columns or want to use the improvements SPP columns offer when high pressure capability isn’t an option to achieve fast and efficient separations. Considering both options can significantly reduce method development time and
help to future proof methods by ensuring the optimum, most efficient, column is being used to reduce re-development needs.
This article is an introduction to the general considerations between FPP and SPP columns, to find out more information about the options available to you or to get guidance on choosing the most suitable column type for your application contact your ELS representative today.
References:
[1] Fekete S and Guillarme, D., 2014. Superficially Porous Particles: Perspectives, Practices, and Trends. LCGC North America.
[2] AMT HALO, HALO – A Better Path to Separation, Available at: About HALO® Columns | Advanced Materials Technology
[3] Dores-Sousa. JL, et al., 2018.Resolving power in liquid chromatography: A trade-off between efficiency and analysis time, Journal of Separation Science, volume 42.
[4] Henderson. J.W., “Plug & Play” Fast and Ultra-Fast Separations Using 3.5-μm Rapid Resolution and 1.8-μm Rapid Resolution High Throughput Columns, Agilent Technologies
[5] Walter, T,H. 2019. Hybrid Particle Columns: The First Twenty Years. LCGC North America.
[6] YMC, (U)HPLC Columns YMC-Triart Brochure.
[7] Majors. R. E (2008) Column Pressure Considerations in Analytical HPLC, LCGC North America.
[8] Superficially porous particles, Element scientific blog, April 2019
[9] Taylor, 2019, “Have we forgotten the advantages of core-shell particles?”
[10] Agilent InfinityLab, Perform Rugged, Fast LC with Confidence Agilent InfinityLab Poroshell 120 columns for HPLC and UHPLC, pg 23